Research
Alzheimer's disease and Extracellular Aβ
Alzheimer's disease (AD) is characterized by two pathologies: amyloid-β (Aβ) plaques and tau neurofibrillary tangles. It appears that Aβ accumulation is the initiating factor that leads to AD, while tau tangles play a critical role in neuronal death and AD progression. Early in disease pathogenesis, soluble Aβ monomer aggregates into soluble Aβ oligomers and insoluble Aβ plaques, both of which cause synaptic dysfunction and kill neurons under certain conditions. Conversion of Aβ from its normal soluble form into these toxic conformations is concentration-dependent; higher concentrations of Aβ are much more prone to aggregate. Amyloid plaques and soluble Aβ oligomers exist within the brain extracellular space. While the initial aggregation seed may form intracellularly or extracellularly, it appears that soluble Aβ within the brain extracellular space, or interstitial fluid (ISF), is one source that contributes to these toxic species. Consequently, maintaining low Aβ levels, particularly within the ISF, should limit creation of toxic forms of Aβ. Our lab focuses on processes that regulate Aβ within the ISF. To do so, we developed unique microdialysis and micro-immunoelectrode (MIE) technologies to measure Aβ specifically within the extracellular space of a living mouse.
Sleep and Aβ

We were the first to demonstrate (when working with Dave Holtzman) that brain Aβ levels fluctuate on a diurnal rhythm with highest levels during wakefulness and lowest levels during sleep. This has been demonstrated several times by our group and others in mice and in humans. Our goal is to define the cellular pathways that link between sleep and Aβ. Orexin is a neuropeptide made exclusively within the hypothalamus which is released throughout the brain to induce wakefulness. Our microdialysis studies demonstrate that during wakefulness orexin activates a phosphatase, SHP2, which dephosphorylates ERK, thus suppressing α-secretase cleavage of APP and increasing brain ISF Aβ levels. We are currently further elucidating this cellular pathway, as well as determining the effect of dynorphin, another neuropeptide co-released with orexin, on Aβ metabolism. Our initial studies suggests that orexin and dynorphin have opposing effects on Aβ levels, despite being released at the same time.
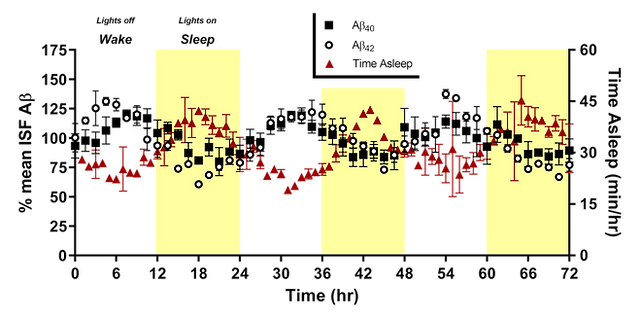
Synaptic-dependent Aβ regulation
Evidence in animal models and humans strongly suggests that neuronal activity regulates amyloid-beta (Aβ) peptide levels and the eventual aggregation as toxic species. Dr. Roberto Malinow’s group was one of the first to directly demonstrate that modulating synaptic activity in brain slice cultures has direct effects on secreted Aβ. Our group then utilized in vivo microdialysis to measure ISF Aβ every hour for several days in awake and freely moving mice. We electrically stimulated the perforant pathway to generate seizures within the hippocampus as measured by EEG (Cirrito et al. 2005). This caused ISF Aβ levels to increase by 30-40% within the first 30 minutes which remained high as long as animals continued to seize. Conversely, blocking synaptic activity with tetrodotoxin (TTX) in vivo reduced Aβ levels by 40%. Many studies, by our group and others, find a direct correlation between the amount of synaptic activity and the amount of Aβ within the brain ISF.
We have determined the cellular mechanism underling this phenomenon in vivo (Cirrito et al. 2008). Synaptic transmission leads to synaptic vesicle recycling which causes more APP to be co-internalized into endosomes by CME. β-secretase and γ-secretase are active within endosomes to cleave APP and produce Aβ. Aβ is then secreted into the extracellular space, or brain ISF. In this mechanism, synaptic transmission drives presynaptic APP endocytosis and Aβ generation. Up to 60% of ISF Aβ formed in the brain appears to rely on this presynaptic, endocytic pathway.
In human brain, regions that have high baseline levels of neuronal activity, for instance the “default mode network”, are also the most vulnerable to developing Aβ plaques. Interestingly, 10% of individuals with temporal lobe epilepsy develop plaques within the hippocampus. Studies in animal models also report that Aβ deposition is also tightly correlated with brain regions with high neuronal activity.
The lab is currently studying the relationship between synaptic activity and Aβ aggregation; does elevated synaptic activity increase Aβ sufficiently, and in the necessary environment, to induce aggregation? We are using the micro-immunoelectrodes (MIEs) to stimulate activity while measuring Aβ monomer and Aβ oligomers in real-time.

Serotonergic regulation of Aβ using SSRIs
Activation of some neurotransmitter receptors leads to altered processing of APP which can impact Aβ generation. Muscarinic acetylcholine receptors activate protein kinase C (PKC) which increases a-secretase cleavage of APP thus reducing Aβ production. Our preliminary data demonstrates that activation of NMDA receptors and serotonin receptors induce second messenger signaling pathways that reduce APP cleavage into Aβ (Cirrito et al. 2011; Verges et al. 2011). For instance, selective serotonin reuptake inhibitor (SSRI) antidepressants rapidly reduce brain ISF Aβ by 25% in mice. And chronic SSRI treatment reduces plaque load. NMDA and serotonin receptors activate the extracellular regulates kinase (ERK) to increase α-secretase activity and reduce Aβ levels. In the case of serotonin, only activation of receptor subtypes 4, 6 and 7 suppress Aβ levels (Fisher et al. 2016). These receptors activate protein kinase A (PKA) to activate ERK and increase α-secretase. Importantly however, not all ligands that activate ERK will suppress Aβ.
The lab is currently studying how serotonin/ERK reduce Aβ, but not other ERK activators, such as norepinephrine or BDNF. We are also collaborating with Dr. Yvette Sheline at University of Pennsylvania to administer SSRIs to cognitively normal controls while assessing CSF Aβ levels over time.

Stress and Sex Differences in Alzheimer’s disease
Results from both human and animal studies over the last decade have identified a clear increase in the rate of Alzheimer’s disease (AD) in women compared to men, even after controlling for life expectancy. The rates of many disorders are different between men and women. Of the disorders that are more prevalent in females, the common underlying feature is an association with stress, such as in anxiety and depression. Stress increases glucocorticoids (GC) in the brain, and the link between GCs and AD has been confirmed by several studies. In AD patients, elevated GC levels are correlated with dementia severity. The female brain is more sensitive to chronic impairment from stress, making the pathways involved in stress particularly important to the differences between men and women in risk for AD. We have previously found that acute stress rapidly increases levels of Aβ (the protein the builds up in the brains of people with AD) in the mouse brain and have recently found that this increase is greater in female mice compared to males. The molecular function of GCs in the brain can happen through 2 different pathways, one that facilitates the activation of certain genes (called transactivation) and another that interferes with other factors to repression gene activation (transrepression). The differences between males and females in transactivation versus transrepression are unclear and the influence of either pathway on AD pathology has not been defined. A mouse model with a mutation that blocks the transactivation function of GCs on their receptor, while allowing effects of transpression has been developed to study differences in these two functions. In order to examine how transactivation or transrepression of GC receptor signaling affects AD pathology, we have crossed these mutant mice (GRdim/dim) with a mouse model of Alzheimer’s disease (APP/PS1). Our preliminary data suggest that blocking transactivation effects of GR signaling escalates AD pathology, particularly in females. The goal of this project is to identify the differences in Aβ production or clearance that drive the exacerbated stress-induced increase in brain Aβ in females, and to identify mechanisms involved in stress signaling that intensify AD pathology in female mice. We hypothesize that stress induces AD pathology and deficits in cognition in female mice greater than in male mice through specific mechanisms of GR signaling. Clarifying the mechanisms underlying these sex-related differences in AD pathology can facilitate the development of more effective treatments.
